Visualizing the dynamic human genome with DHO 3D tracking and Light Sheet Microscopy
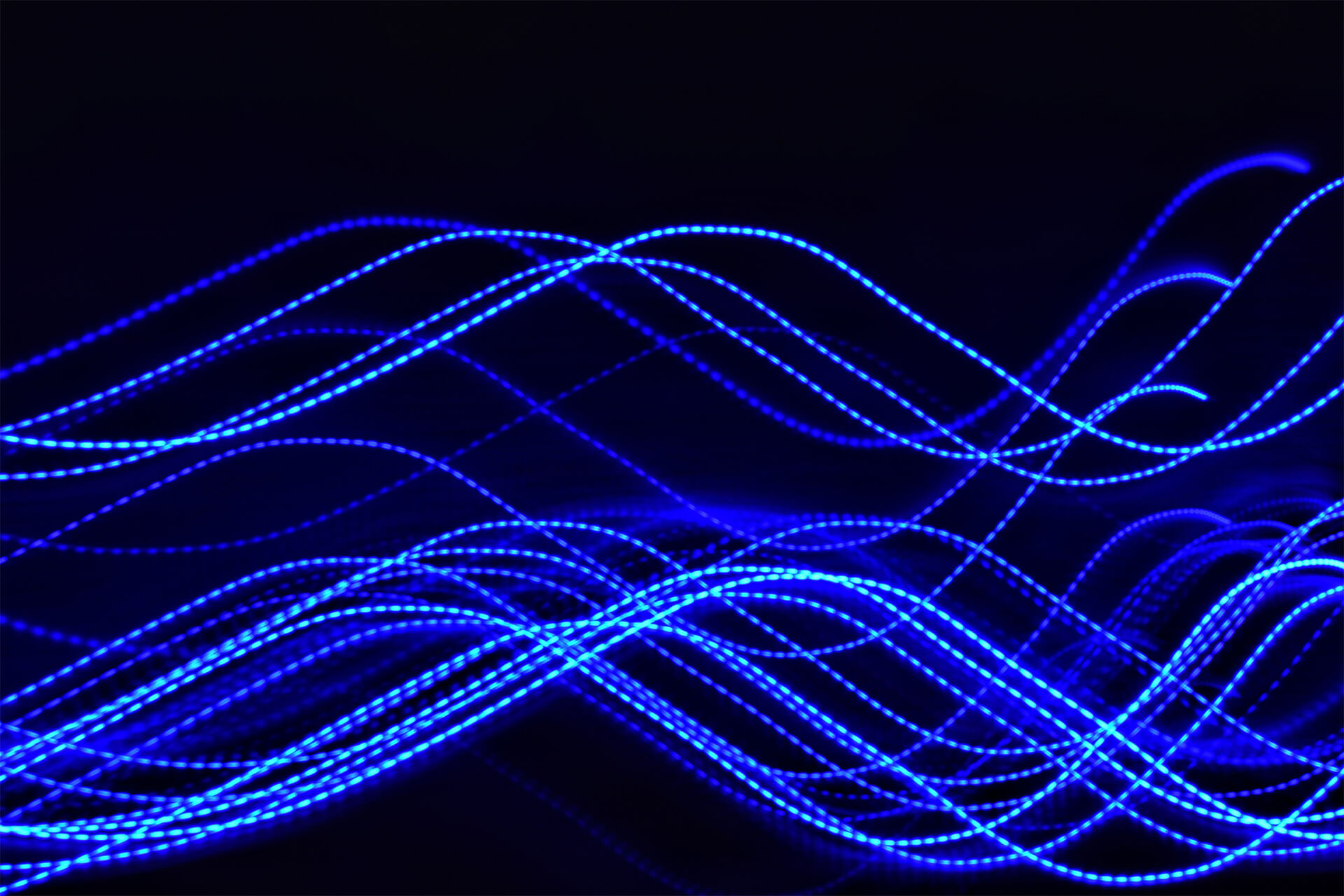
Using a DHO double-helix phase mask combined with light sheet microscopy, the Gustavsson Lab at Rice University has developed a new way to monitor chromatin throughout the entire nucleus over long time scales, revolutionizing our ability to understand its dynamics and changes in response to disease.
Why this is important
The human genome is a vast and highly organized entity within the cell nucleus, and this ever-changing architecture is essential to its function. Despite the grand achievement that was sequencing the human genome, this is just the beginning of understanding its inner workings. The key to deciphering the genetic code is centered on its organization: How does the location of a gene relative to other genes and elements in the nucleus regulate its activity? There is a great amount of evidence supporting the existence of a compartmentalized nature to the nucleus. The advancement of knowledge of this phenomenon will help answer biophysical and biochemical questions relevant to aging and diseases like cancer.
Such a task requires the development and application of advanced imaging methods, which is the focus of the Gustavsson group. This led them on a path to creating a novel 3D single molecule technique to follow chromatin to all corners of the nucleus.
The science
Imaging chromatin throughout the entire nucleus over long timescales presents optical challenges. The fluorescent tags on these genes need to be both stable and very bright. The Gustavsson group solved this through the utilization of nanobodies that are capable of penetrating the nucleus. When fused to Cas9, these nanobodies could be targeted to specific DNA sequences while bound to green fluorescent protein (GFP) molecules that could be replenished over the course of the long experiment. Additionally, it is difficult to image a large sample such as the mammalian nucleus with an acceptable signal-to-noise ratio while also limiting photo damage when illuminating all at once. Light-sheet microscopy however allows for good contrast and a gentle treatment of the cell. The Gustavsson group previously optimized a tilted light sheet design (TILT3D) for 3Dsingle-particle tracking on a light-sheet microscope.
Using these advancements, multiple chromosomal loci were tracked in parallel in three dimensions throughout the nucleus over thousands of frames. Importantly, sample drift and nuclear movement was accounted for by using fiducials in 3D. This complete collection of data provided rich information about the local chromatin dynamic behavior and re-organization events that underly important biological processes. Specifically, the Gustavsson group assessed the global effects of actin polymerization on the diffusion speed of chromatin. After inhibiting polymerization, they observed a global increase in diffusion speeds of chromatin and a change to a more isotropic (even in all directions) nature.Additionally, they found a significant heterogeneity in their data, an emerging subject on the topic of cancers and the cell cycle.
How Double Helix Optics made it possible
Until recent technological advancements, 3D chromatin architecture has primarily been studied using sequence and proximity-based methods. These techniques provided single time point snapshots of organizational information and required harvesting biomolecules from lysed cells. Greater details of this process can be attained spatially and in the context of live cells with single molecule microscopy techniques. 2D imaging techniques like widefield and TIRF imaging were capable of improving upon this standard in the field but required sequential acquisition of z-slices. These tracking techniques are incomplete because motions can be missed if that slice isn’t being imaged at a particular moment. 3D imaging is uniquely able to observe processes that are dynamic and have a z-component, which is almost always the case inside cells. The Gustavsson Lab employed 3D single particle tracking (3D-SPT) using the double-helix point spread function (DH-PSF) with light-sheet microscopy to form a cutting-edge approachable to monitor long-term actions across ranges up to 10 µm thick.
A double helix phase mask was used as an upgrade with an IX71 Olympus inverted microscope at the Rice University Department of Chemistry.